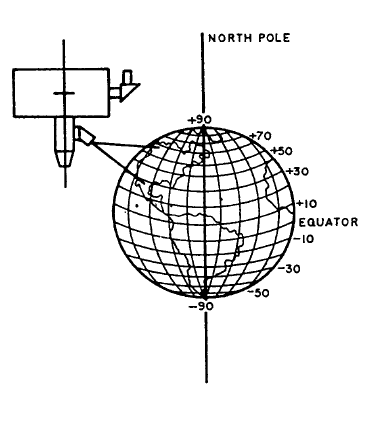
10
The celestial meridians of longitude are called
"coordinates of right ascension (R.A.)", and like the
Earth's longitude meridians they extend from pole to
pole. There are 24 major RA. coordinates, evenly
spaced around the 360º equator, one every 15º. Like
the Earth's longitudes, R.A. coordinates are a measure
of time as well as angular distance. We speak of the
Earth's major longitude meridians as being separated
by one hour of time because the Earth rotates once
every 24 hours (one hour = 15°). The same principle
applies to celestial longitudes since the celestial sphere
appears to rotate once every 24 hours. Right
ascension hours are also divided into minutes of arc
and seconds of arc, with each hour having 60 minutes
of arc and each arc minute being divided into 60 arc
seconds.
Astronomers prefer the time designation for R.A.
coordinates even though the coordinates denote locations on the celestial sphere, because this makes it
easier to tell how long it will be before a particular star will cross a particular north-south line in the sky.
So, R.A. coordinates are marked off in units of time eastward from an arbitrary point on the celestial
equator in the constellation Pisces. The prime R.A. coordinate which passes through this point is
designated "O hours O minutes O seconds". We call this reference point the vernal equinox where it
crosses the celestial equator. All other coordinates are names for the number of hours, minutes and seconds
that they lag behind this coordinate after it passes overhead moving westward.
Given the celestial coordinate system, it now becomes possible to find celestial objects by translating their
celestial coordinates using telescope pointing positions. For this you use setting circles for R.A. and Dec. to
find celestial coordinates for stellar objects which are given in star charts and reference books.
Polar Alignment
Polar alignment is the process by which the telescope’s axis of rotation is aligned (made parallel)
with the Earth’s axis of rotation (see figure 7). Once aligned, a telescope with a motor drive will
track the stars as they move across the sky. The result is that objects being observed through the
telescope appear stationary (i.e., they will not drift out of the field of view). If your telescope does
not use a motor drive, all celestial objects in the sky (day or night) will slowly drift out of the field.
This motion is caused by the Earth’s rotation. Even if you are not using a motor drive, polar
alignment is still desirable since it will reduce the number of corrections needed to follow an object
and limit all corrections to one axis (R.A.). There are several methods of polar alignment, all of
which work on a similar principle, but are performed somewhat differently.
For each hemisphere, there is a point in the sky around which all the other stars appear to rotate.
These points are called the celestial poles and are named for the hemisphere in which they
reside. For example, in the northern hemisphere all stars appear to move around the north
celestial pole (see figure 6). When the telescope’s polar axis is pointed at the celestial pole, it is
parallel to the Earth’s rotational axis.
Many of the methods of polar alignment require that you know how to find the celestial pole by
identifying stars in the area. For those in the northern hemisphere, finding the celestial pole is not
difficult. Fortunately, we have a naked eye star less than a degree away. This star, Polaris, is the
end star in the handle of the Little Dipper. Since the Little Dipper (technically called Ursa Minor) is
not one of the brightest constellations in the sky, it may be difficult to locate, especially from urban
areas. If this is the case, use the two end stars in the bowl of the Big Dipper. Draw an imaginary
line through them toward the Little Dipper. They will point to Polaris. The position of the Big
Figure 5